Is there a fuel cell in the automobile's future?
A major challenge facing the Automotive Industry is the development of an affordable "Supercar" that has drastically lower fuel consumption. One of the goals defined by the Partnership for a New Generation of Vehicles (PNGV) calls for a production-prototype car that has comparable range, performance, utility and safety with today's midsize sedan and yet obtains up to three times higher fuel economy
April 1, 1996
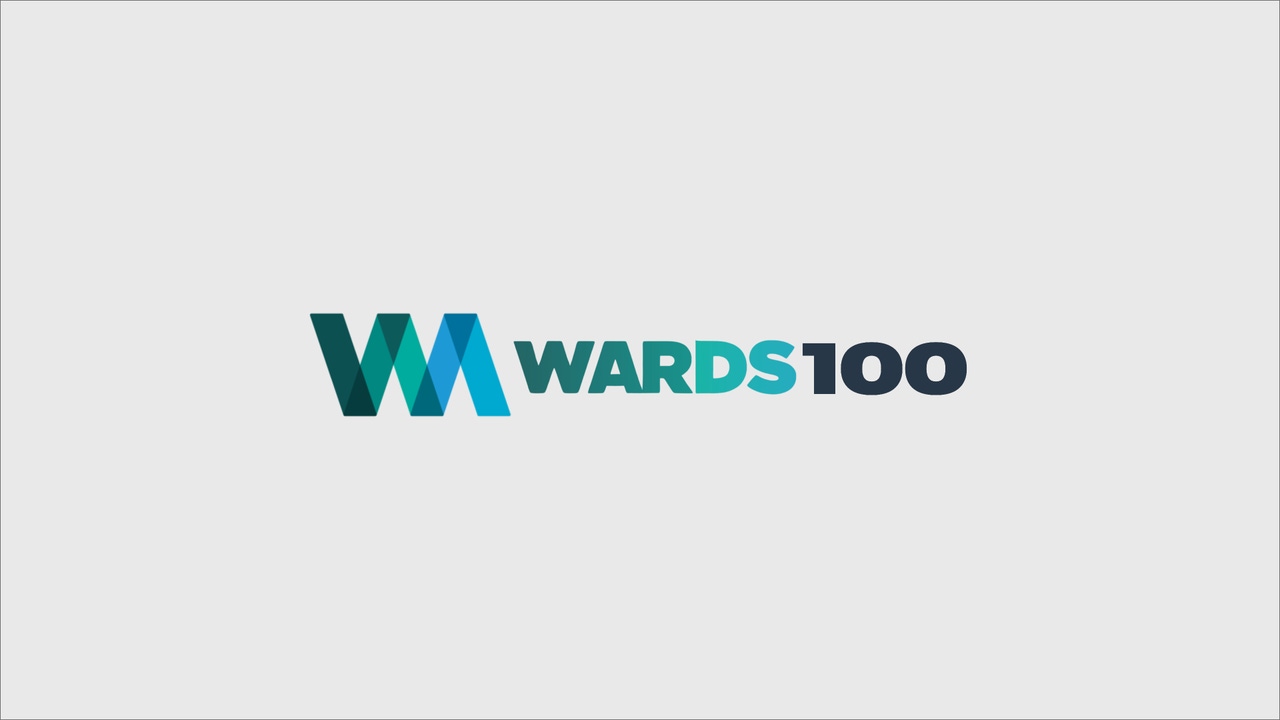
A major challenge facing the Automotive Industry is the development of an affordable "Supercar" that has drastically lower fuel consumption. One of the goals defined by the Partnership for a New Generation of Vehicles (PNGV) calls for a production-prototype car that has comparable range, performance, utility and safety with today's midsize sedan and yet obtains up to three times higher fuel economy (80 mpg) and produces Tier Il level emissions.
The quest for this "Supercar" began in 1993 with the creation of PNGV, an historic alliance between the federal government (represented by seven agencies) and the Big Three (represented by USCAR). PNGV has set up 10 technical teams to help manage the research of key advanced technologies needed to develop the "Supercar" project. The three principal energy-conversion technologies currently being developed and managed by the Big Three are fuel cells, direct-injected compression ignition engines and gas turbines. Fuel cells may not be commercially viable in the 2004 timeframe set by PNGV, but their potential to solve a wide range of environmental issues promises to keep them under consideration by all automakers for the foreseeable future.
Fuel cells create electricity in a chemical reaction between hydrogen and oxygen (from the air) at ambient temperature and without necessarily generating pollution. the fuel cell looks like a battery, but the key difference is that a battery stores energy in its electrodes whereas fuel cells, like heat engines, receive stored energy from a fuel tank. This decoupling of energy (range) and power (performance) allows the fuel cell to offer, potentially, the best of both worlds: a long-range, rapidly refueled, onboard zero-emission vehicle.
As with batteries, a fuel-cell stack contains several hundred cells that each produce around one volt. Each cell contains a polymer film electrolyte, called a Proton Exchange Membrane (PEM), and two electrodes (an anode and cathode) that must be coated with a platinum catalyst in order for the necessary reactions to occur at ambient temperature. The electrodes resemble carbon paper, and the resulting membrane-electrode assembly is sandwiched between two bipolar plates to create each cell. These plates, a few millimeters thick, are currently machined from graphite with channels for directing the hydrogen and air into the cells and for removing the by-product water. Their other function is to conduct current from cell to cell, which enables useful stack power to be built up for energizing a traction motor to turn the wheels.
Recent interest in fuel cells has emerged in response to the 60-fold reduction in platinum content since 1984.
The significance of this is that there may now be no inherently expensive material costs in the fuel cell. However, if fuel cells are mass produced using today's techniques they would cost over $200/kW, whereas a conventional powertrain costs under $30/kW. Since the major cost-driver in fuel cells is currently the bipolar plate, corrosive-resistant coated steels or conducting plastics are being developed because they could use low-cost stamping or injection-molding processes.
Since 199 1, fuel-cell power density has increased seven-fold to over IKW/L. Volume should no longer be regarded as a major concern for automotive applications, particularly since modularity and shape flexibility may allow a 50 kW fuel-cell stack to be placed down the floor tunnel of an existing midsize sedan.
The primary reason that fuel cells are being considered is their potential for high efficiency. A gasoline engine is about 20-25% efficient over the test drive cycle; fuel cell systems, including all their parasitic losses, have demonstrated 45-50% efficiency under similar conditions, if they use hydrogen.
Here lies the second problem facing fuel-cell commercialization, and this might be even more serious than die cost challenge. Even if hydrogen storage and safety are, generously, given the benefit of the doubt, the lack of a hydrogen infrastructure still forces automakers to consider less-attractive alternatives (from the fuel cell's perspective). If other fuels are used, such as gasoline, then they must first be converted (or reformed) into hydrogen on-board the vehicle before the hydrogen can be fed into the fuel cell. Even though reforming is a gentler process than combustion, it still introduces trace emissions, and the overall efficiency is dragged down.
Ironically, hydrogen gives fuel cells their unique properties but is also the major obstacle to their commercialization. If fuel cells are to be practical in the near-term, then efficient ways to process fossil fuels on-board a vehicle must be demonstrated, and costs must be slashed from the fuel-cell stack itself. Ultimately, renewable energy costs will fall, hydrogen should become more attractive, and the fuel cell could become the logical successor to the internal combustion engine.
About the Author
You May Also Like